My Biophysics
Kazuhiko KINOSITA, Jr.
Center for Integrative Biosicence
Okazaki National Research Institutes
November, 2000
Biology was the subject that I disliked most, until I had
almost graduated from the physics department of a university some 30 years
ago. In those days, biology at school was nothing but an act of
extensive memorization, like listing the numbers of petals and stamens. It
was one electron micrograph that opened my eye to biological science, the
photograph that served as the basis of the sliding theory of muscle contraction
( Fig. 1). A muscle is made of thick and thin
filaments that interdigitate against each other. Sliding of the
two sets of filaments past each other causes the muscle to contract. OHHHHH,
I seeeeee, ... I got it!! For the first time I saw, in the realm
of living beings, an 'explanation,' which was so clear-cut. And
it was an explanation that I could never have thought of.
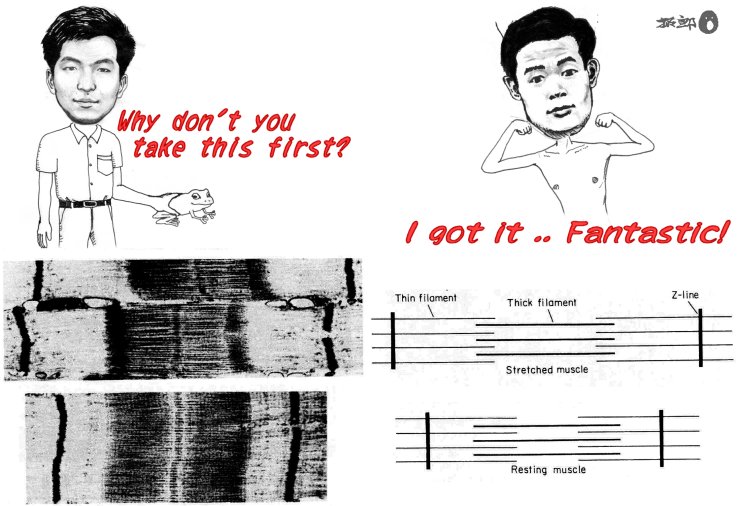
|
Fig. 1. Sliding
mechanism of muscle contraction. Lower left, electron micrographs
by Dr. H. E. Huxley. Lower right, schematic diagram. A
muscle is comprised of thick and thin filaments, made of myosin and actin
molecules, respectively. Contraction results from the mutual sliding
of the two sets of filaments against each other, while the filament lengths
remain constant. Comparison of the upper (before contraction)
and lower (after contraction) micrographs reveals that the length of the
thick filaments indeed remained constant.
|
So beautiful was the explanation that I was immediately
convinced that it must be so. But I was still an amateur at that
time; now, I would not believe in any theory so easily. Believing
belongs to religions, in my opinion. If a scientist says that
he/she believes some explanation, that, I 'believe,' is nothing but a confession
of the absence of concrete evidence. If a person says (and really
believes) that something must be absolutely true, that person is not a 'true'
scientist, in my view.
The person who introduced the electron micrograph in Fig. 1 to me was Mr. I, who remains as one of my favorite colleagues and best friends. Mr. I
tempted me to participate in an experiment, to be presented at a college
festival, using a frog muscle. I am the kind of person who cannot
touch a frog (though I can hold a rabbit). Nevertheless, the frog
experiment gave me a high and eventually put me on the track toward becoming
an experimental biophysicist. Mr. I operated on frogs,
while my role was to build a recording instrument. This casting
has since been fixed for 30 years or so. Without Mr. I, I would not have entered the field of biophysics.
During my teens, I was fascinated by physics, because of
its simple and universal way of explaining many things. After
entering a university, I soon realized that mastering physics wouldn't be
that simple. I chose biophysics as the escape, which, in retrospect,
was not a bad choice. My original hope was to find an explanation
or two, of my own, in the world of living things. Unfortunately,
this hope never materialized while I was an experimentalist.
Small technical ideas are often rewarded in experiments,
and most experiments produce some data, meaningful or not. Thus,
I never got tired of an experiment. And, I forgot the holy purpose
of explaining something. I did try, occasionally, to invent an
explanation, but proving/disproving the imaginary idea was beyond my experimental
ability. I reconciled myself to being a measurer, wishing some
of my data might turn out to be of some use to someone. I ended
up inhabiting a nook of the world of mere descriptions, which I hated at
the beginning.
The age came at which I got a position that allowed me
to beg students to come, and I found myself losing energy for sustained concentration
on an unrewarding experiment. Quite regrettably (and unexpectedly!),
young students proved to perform much better than myself. Then
I realized that all I needed to do was to ask the great students to do important
experiments that could lead to an explanation. It's so easy to
ask a person, other than myself, to try a difficult task that is apparently
far beyond the ability of that person. That this works, that cajoling
(deceiving!) makes impossible possible, was taught to me by Mr. Y. Not
that he told me the secret. I simply learned this trick myself,
by looking at a marvelous achievement made by a student of Mr. Y. Deceiving
oneself is not easy. I tell students and my young colleagues that
they should make an explicit effort at being deceived naively, or to 'believe'
in possible (probable!) success.
A couple of young 'others' (myself not included) demonstrated
the existence of a rotary motor made of a single protein molecule. They
attached a long rod to the putative rotor subunit of the protein molecule,
and then the rod rotated under an optical microscope, under my eyes ( Fig. 2). The
rotation was so beautiful that I instantly felt it must be 'almost true.' Utmost
joy would it have been, had it been myself who did the experiment. So
jealous was I, that I never touch this motor myself. The cartoon
in Fig. 2 is fake.
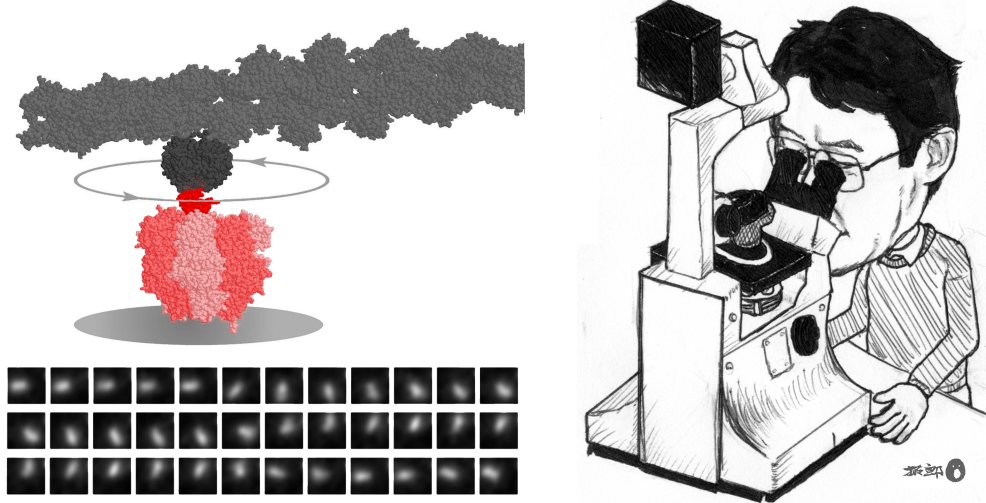
|
Fig. 2. F1-ATPase,
a rotary motor made of a single protein molecule. Of the red complex
which represents the motor molecule, the protrusion at the top, painted deep
red, rotates against the surrounding units. To prove the rotation,
my young colleagues attached an actin filament (the gray, horizontal rod
at the top; its length was ~100 times the size of the motor) to the putative
rotor. As seen in the sequential micrographs at the bottom, the
rod rotated counterclockwise in discrete 120° steps. The F1-ATPase turned out to be a stepper motor. In our body, numerous F1 motors rotate in the reverse direction, to synthesize ATP, the energy source of all our activities. |
I would like to ask 'others' in my neighborhood to work
out the explanation of how this rotary motor operates. I hope
they will also elucidate the mechanisms of various other biological molecular
machines one after another. I think that molecular machines work
by changing their conformations: by bending, stretching, or twisting their
body. The causes, and also consequences, of the conformational
changes are association with, or dissociation from, other molecules (small
ligands such as ATP or another protein molecule), electrical potential, light,
etc. Visual explanation of the interactions and conformational
changes, in the form of movies, is our prime goal.
After talking with many scientists, I realize that the
kind of explanation that I want is always 'how.' In contrast,
most biologists seem to seek an answer in the form of 'who does what.' Naming
appears to be their prime job. For example, it is Rhodopsin that
absorbs light in the eye, Transducin is the protein that receives a signal
from the activated Rhodopsin, and Phosphodiesterase in turn is activated
by Transducin, ...... Biologists search for actors who play distinct
roles in biological activities, name them (to claim that they really exist
and that they are each distinct from all others), and describe their role
(character) in one short phrase. Is this mere refinement of the
listing of the numbers of petals and stamens? No. Entirely
different. Identification of distinct actors (characters) in a
complicated play is by itself an excellent explanation.
But, nevertheless. I would like to inquire into
how individual actors actually play: how they stand, how they dance, how
they sing, and, above all, how they obtain power. Reading the
cast of characters alone is far from satisfying. Many biophysicists
seem to share the same feeling. Biophysicists get bored when they
listen to many names in a lecture, whereas cell biologists get bored if they
hear only a few names (supplemented with equations). Explaining
and discussing the physical mechanism of one rotary motor for one hour before
cell biologists should be avoided.
If one is to understand how an actor made of a single molecule
behaves, one has to watch individual molecules. Molecules are
exposed to, and they themselves undergo, extensive thermal (Brownian) motions. As
such, their performances are necessarily stochastic; multiple molecules never
perform in synchrony. Thus, macroscopic measurements, which report
on the average behavior, usually fail to reveal the details of the performance.
The average speed of the thermal motion of water molecules
is about 1,000 km per hour, the speed of a jumbo jet, and is close to the
speed of sound in air. If a protein molecule were of your size,
a water molecule would be a golf ball, or a bullet of a gun, both in size
and weight, roughly. Imagine you are hit by numerous golf balls,
or a hail of bullets, at speeds exceeding that of a bullet from a real gun. Protein
machines perform their functions in such a merciless environment. Don't
you agree these admirable performers deserve full description? It
will be a pity if they are known only by name. I want to watch
them as closely as possible.
In our lab, optical microscopes are the only tool of observation. Although
their spatial resolution is not as high as that of electron microscopes,
there is the merit that one can continuously observe, and take a movie of,
a single molecule while it is performing its function. As seen
in Fig. 2, molecular machines perform well even when
they carry a tag that is hundreds of times as large as their own body. Such
a huge tag reveals details of the molecular performances under an ordinary
microscope. Or, a small tag such as a single fluorophore can be
attached at a desired position in the machine, and it will report on the
movement of that particular part. In addition to the observation
using huge and small tags, we can manipulate individual molecules with optical
and magnetic tweezers. The term 'Single-Molecule Physiology' best
describes our endeavors.
Let me conclude this essay with Fig. 3. The
sliding theory of muscle contraction has been accepted by many, though not
all, researchers. But the mechanism by which myosin slides past
actin has remained unclear. Now the electron micrographs in Fig. 3
suggest that the two-legged myosin (a relative of muscle myosin) may literally
walk, by the alternate use of the two legs. The way it leans forward
by bending its fore ankle appears to be that of a human. These
micrographs, which appeared in the journal Nature this year, gave me a jolt,
as the Huxley micrographs did 30 years ago. This time, the explanation
was an already anticipated one, but seeing these images was really impressive. Was
seeing believing? Well, I am now a professional scientist, and
thus I am not yet convinced that myosin walks as a human does.
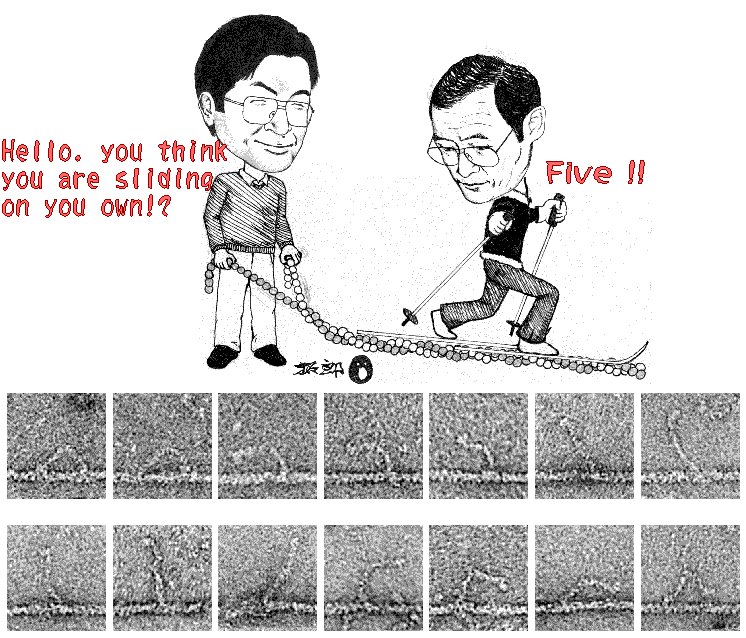
|
Fig. 3. Myosin
V, a member of myosin family, may 'walk,' using its two legs, according to
P. J. Knight and colleagues who presented these electron micrographs (Nature, 405 (2000) 804-807). These
are not the sequential snap shots of the movement of the same molecule, and
are arbitrary selections from a collection of independent images. Nevertheless,
they give an impression that myosin V really walks. I particularly
like the second image, where myosin V takes the telemark position, leaning
forward by bending its fore leg. The horizontal rod, which looks
like a twisted pearl necklace, is an actin filament on which myosin V moves. The
possibility that the actin filament is the principal actor driving myosin,
rather than being a passive track, cannot be dismissed entirely.
|
|